Wind energy accounts for an increasing share of the energy mix thank to the construction of wind farms designed to reduce investment and operating costs. However, within a farm, downstream wind turbines are exposed to the wakes of upstream rotors, leading to a reduction in their production and an increase in their fatigue stresses. Moreover, wind turbines operate in a complex environment, known as the atmospheric boundary layer (ABL), which interacts with these wakes. This zone of the atmosphere is subjected to large-scale effects (pressure gradients, Coriolis effectsa) as well as local effects, such as thermal exchange with the ground and the surrounding topography.
For numerical simulation (as illustrated in Figure 1), a real challenge exists, associated with the multi-scale nature of the problem: it is necessary to analyze and model the impact of kilometric phenomena (atmospheric turbulence) on wakes, the origin of which is on a metric scale (flow around a blade).
To address this problem, a research partnership was set up with the CNRM (French National Meteorology Research Center) hinged around the Meso-NH simulation tool. The CNRM integrated precise ABL modeling into the tool, including the various phenomena of importance for wind energy technology, such as turbulence, thermal stratification and topography[1]. For its part, IFPEN implemented representative wind turbine models in the tool, based on actuator line and disc approaches [2] [3].
As a result of these changes, researchers were able to use Meso-NH to study wake behavior [4][5][6] and develop analytical models that have since been incorporated in FarmShadowTM, the wind farm design tool developed by IFPEN.
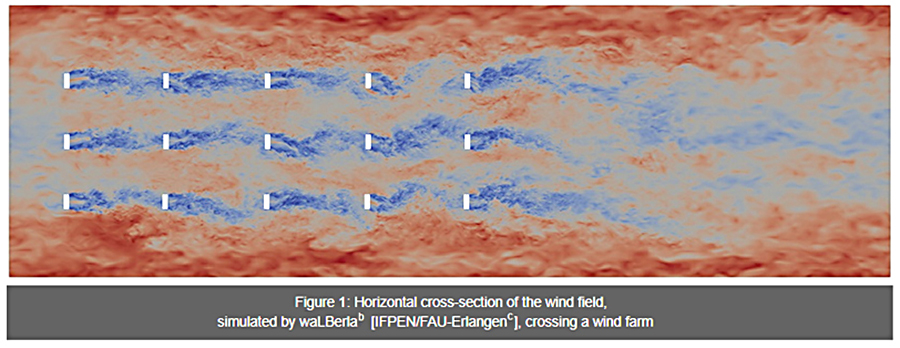
The resulting improvement compared to existing simulation tools is that FarmShadowTM makes it possible, in a few seconds of calculation time, to estimate the production of wind farm. Combined with IFPEN’s optimization methods, it also makes it possible to maximize a farm’s overall production by adjusting wind turbine placement.
Moreover, new analytical models of unsteady wakes interacting with the ABL will be employed in the DeepLines WindTM aero-hydro-servo-elastic computational code, used to design a wind turbine within a farm.
Regarding high-fidelity simulations, one sticking point still to be overcome is that of calculation time: conducted by LESd, simulations arelimited from a practical point of view to a few configurations including only a few turbines. An alternative approach, based on Lattice Boltzmann methods (LBM), may make it possible to overcome this challenge and is currently the focus of research (figures 1 and 2), in partnership with Erlangen University [7]. By exploiting the capacities of computer graphics cards, early results show a 400-fold reduction in calculation time. Work is in progress to implement in this solver the physical models necessary for the simulation of CLA.
a- Coriolis force: an inertial force that acts perpendicular to the direction of movement of objects in motion in a medium itself rotating uniformly
b- waLBerla: Widely applicable Lattice Boltzmann from Erlangen: massively parallel framework for multi-physical applications
c- FAU-Erlangen: Friedrich-Alexander-Universität Erlangen-Nürnberg
References:
- Lac, C., Chaboureau, J. P., Masson, V., Pinty, J. P., Tulet, P., Escobar, J., ... & Wautelet, P. (2018). Overview of the Meso-NH model version 5.4 and its applications. Geoscientific Model Development, 11(5), 1929-1969.
>> DOI: 10.5194/gmd-11-1929-2018
- Joulin, P. A. (2019). Modélisation à fine échelle des interactions entre parcs éoliens et météorologie locale (Doctoral dissertation).
>> https://www.theses.fr/2019INPT0135
- Joulin, P. A., Mayol, M. L., Masson, V., Blondel, F., Rodier, Q., Cathelain, M., & Lac, C. (2020). The actuator line method in the meteorological LES model meso-NH to analyze the horns rev 1 wind farm photo case. Frontiers in Earth Science, 7, 350.
>> DOI: 10.3389/feart.2019.00350
- Blondel, F., Cathelain, M., An alternative form of the super-Gaussian wind turbine wake model, Wind Energ. Sci., 2020
>> DOI: 10.5194/wes-5-1225-2020
- Blondel, F., Cathelain, M., Joulin, P.A., Bozonnet, P., An adaptation of the super-Gaussian wake model for yawed wind turbines, J. Phys.: Conf. Ser. 1618 062031, 2020
>> DOI: 10.1088/1742-6596/1618/6/062031
- Jézéquel, E., Blondel, F., and Masson, V.: Breakdown of the velocity and turbulence in the wake of a wind turbine – Part 2: Analytical modeling, Wind Energ. Sci. Discuss. [preprint], in review, 2022
>> DOI: 10.5194/wes-2022-47
- Schottenhamml, H., Anciaux-Sedrakian A., Blondel F., Borras-Nadal, A., Joulin, P.A., Rüde, U., Evaluation of a lattice Boltzmann-based wind-turbine actuator line model against a Navier-Stokes approach, 2022
>> DOI: 10.1088/1742-6596/2265/2/022027
Scientific contacts: pierre-antoine.joulin@ifpen.fr ; frederic.blondel@ifpen.fr