For around fifteen years now, IFPEN has been focusing on modeling conventional batteries to represent their nominal operation (electric and thermal behavior during normal operation), throughout their lifetime [1-2] (evolution of performance associated with degradation mechanisms) and in the event of thermal runaway (failure, improper use) [3–5].
Battery technologies, for instance lithium-based, are constantly evolving in order to increase their energy density, reduce their cost and improve their operational safety. At the same time, these multiple evolutions require continual adaptation in terms of the naturea and structureb of the models used to describe their behavior.
One of the best examples of these evolutions concerns the significant volume variations of electrode materials, during charge and discharge cycles. These lead to variations in internal mechanical stresses and changes in the interfaces between components, with an impact on battery performance. This is the case for high-performance batteries in which the negative electrode contains siliconc and for which this mechanical dimension needs to be added to the multi-physical and multi-scale models previously developed [2]. This is the aim of the European MODALISd project (figure), coordinated by IFPEN, and the PSPC-Régionse Auranode project, conducted in partnership with Enwires. The new 4th generation of lithium batteries, also known as “all-solid-state” batteriesf, is also concerned by these evolutions. This “all-solid-state” generation will be studied within the framework of the European HELENAg project, in which IFPEN is leading modeling activities.
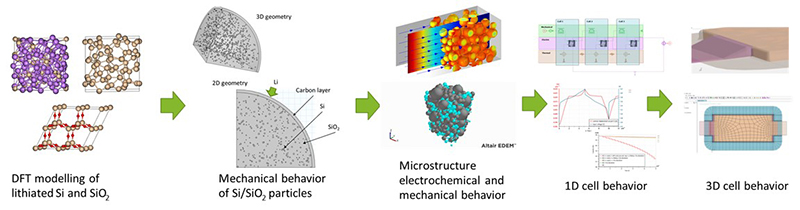
Models can also be enhanced by better consideration of the mechanisms at electrode/electrolyte interfaces in these different systems (liquid/solid or solid/solid interface). The scale of the reactions at these interfaces requires the use of molecular modeling approaches, which is the focus of PhD research conducted in partnership with ENS Lyon engineering school and Stellantis.
Moreover, improving the safety of new battery technologies requires the modeling of phenomena that can cause thermal runaway or system degradation. The model developed in IFPEN describes the exothermal degradation reactions affecting the battery components. It can simulate the cell’s temperature, voltage and internal pressure evolution during thermal runaway. It can also be used to study the propagation of this thermal runaway between the neighboring cells in a battery pack. Lastly, it is coupled with an aging model, so that the impact of aging on battery thermal stability can now be taken into account. [6].
For all these developments carried out by IFPEN, a multi-physical and multi-scale approach is used based on combining electrochemistry with diffusion, interfacial, thermal and mechanical phenomena. This approach is already used to study other energy storage systems, such as flow batteries, or fuel cells [7].
a- Electrical, electrochemical, mechanical, chemical
b- Coupled/uncoupled, complex/simplified
c- Generation 3b (high capacity/high voltage)
d- MODelling of Advanced LI Storage Systems
e- Strategic research and development project for competitiveness
f- In which lithium is used for the negative electrode and where the liquid electrolyte is substituted with a solid electrolyte
g-Halide solid state batteries for electric vehicles and aircrafts
References:
- Edouard C., Petit M., Bernard J., Forgez C., Revel R. (2015) Sensitivity Analysis of an Electrochemical Model of Li-ion Batteries and Consequences on the Modeled Aging Mechanisms, ECS Transactions 66, 9, 37–46.
>> DOI: 10.1149/06609.0037ecst
- Petit M., Calas E., Bernard, Julien, Julien (2020) A simplified electrochemical model for modelling Li-ion batteries comprising blend and bidispersed electrodes for high power applications, J.Power Sources 479, 228766.
>> DOI: 10.1016/j.jpowsour.2020.228766
- Nguyen T.T.D., Abada S., Lecocq A., BERNARD J., Petit M., Marlair G., Grugeon S., Laruelle S. (2019) Understanding the Thermal Runaway of Ni-Rich Lithium-Ion Batteries, WEVJ 10, 4, 79.
>> DOI: 10.3390/wevj10040079
- Abada S., Petit M., Lecocq A., Marlair G., Sauvant-Moynot V., Huet F. (2018) Combined experimental and modeling approaches of the thermal runaway of fresh and aged lithium-ion batteries, Journal of Power Sources 399, 264–273.
>> DOI: 10.1016/j.jpowsour.2018.07.094
- Petit M., Abada S., MINGANT R., BERNARD J., DESPREZ P., PERLO P., BIASIOTTO M., INTROZZI R., Lecocq A., Marlair G. (2019) Demobase project: Numerical simulation for seamless integration of battery pack in light electric vehicle.
>> https://zenodo.org/record/3368893
- IFPEN (2022) IFPEN | La modélisation pour améliorer la sécurité des batteries lithium-ion. Available at:
>> https://www.ifpenergiesnouvelles.fr/article/modelisation-ameliorer-securite-des-batteries-lithium-ion
- Cacciuttolo Q., Petit M., Pasquier D. (2021) Fast computing flow battery modeling to optimize the choice of electrolytes and operating conditions – application to aqueous organic electrolytes, Electrochimica Acta 392, 138961.
>> DOI: 10.1016/j.electacta.2021.138961
Scientific contacts: sara.abada@ifpen.fr ; martin.petit@ifpen.fr ; julien.bernard@ifpen.fr