28.04.2021
4 minutes of reading
Finding substitutes for products of fossil origin necessarily implies optimizing and managing processes that use a renewable raw material. This is the case, for example, in the production of butadiene, a compound widely used to make synthetic rubber. Revisiting an old process that starts with ethanol thanks to a better understanding of the reaction mechanisms involved, IFPEN’s researchers have defined an original scheme, combining the hitherto generally accepted Gorin-Jones route with a reaction route known as the “Inoue route”. The scheme has been shown to be relevant for explaining the composition of effluents associated with the Ostromislensky catalytic conversion process, paving the way for future process improvements.
Butadiene is a monomer of industrial interest used in the synthesis of different polymers, such as Polybutadiene Rubber (PR), Styrene-Butadiene Rubber (SBR) and Acrylonitrile Butadiene Styrene (ABS). It is the only compound from the family of C4-hydrocarbons to be exclusively used in petrochemical production, particularly for the production of synthetic rubber. This makes it a key ingredient for the tire industry.
Biobased butadiene production, the result of a complex conversion process
On an industrial scale, butadiene is traditionally produced in steam cracker units, from fossil hydrocarbons. However, market needs and the drive to reduce reliance on oil require research efforts to find an alternative solution. The solutions currently being explored include processes that use bioethanol as a raw material. One of these is the Ostromislensky process, consisting of a sequence of two catalytic reactions, each taking place in a dedicated reactor1.
A whole series of separation steps is then necessary, because this complex conversion generates an effluent composed of numerous secondary oxygenated and hydrocarbon compounds. Therefore, optimizing the biobased butadiene production process hinges around the understanding and control of the reaction mechanisms at play.
Alongside the Gorin-Jones reaction route, IFPEN proposes the Inoue route
IFPEN’s researchers conducted a study to establish an original reaction scheme describing this reaction characterized both by a significant industrial interest and and by a sustainability challenge [1]. Until now, the reaction route underpinning butadiene production widely accepted in the literature was the Gorin-Jones route [2-3]. However, the latter did not make it possible to explain the formation of compounds with an odd carbon number.
It is for this reason that the authors of the IFPEN study proposed to add a reaction route involving the transfer of intramolecular hydrogen between reaction intermediates, called the “Inoue route” [4]. They also supplemented the global reaction scheme (figure 1) with additional reactions to explain the formation of major (ethylene, diethyl ether, ethyl acetate) and minor (olefins, C4 oxygenated compounds and heavier compounds) secondary compounds.
This reaction scheme was partly established on the basis of tests conducted on a laboratory unit, using dedicated molecules, to validate their reactivity and verify the accuracy of the selected reaction routes.
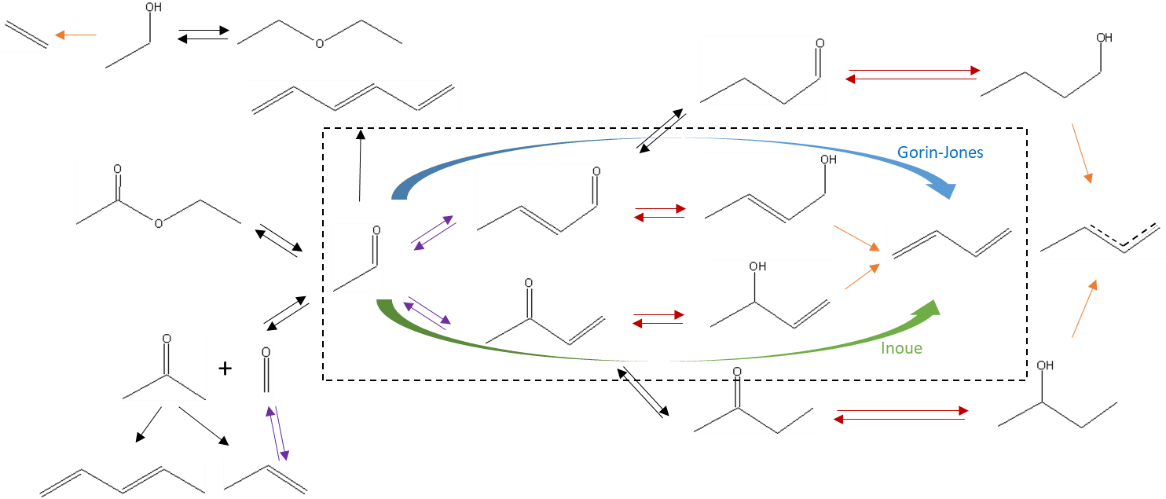
IFPEN’s researchers then integrated this reaction scheme, associated with kinetic laws, into a reactor model making it possible to predict the composition of effluents from the reaction section as a function of operating conditions: temperature, pressure, flows, ethanol/acetaldehyde ratio in the feed. They estimated the parameters of the kinetic model by referring to a dedicated experimental data base, acquired via the use of a high-throughput experimentation unit for a broad range of operating conditions2.
A relevant reaction scheme for new perspectives
Comparing the simulated data using the kinetic model developed in this study with experimental results (figures 2 and 3) demonstrates the relevance of the reaction scheme combining the Gorin-Jones and Inoue routes proposed for butadiene production. The model accurately reproduces the carbon mass flows associated with the main compounds (ethanol, acetaldehyde, butadiene), the intermediate carbonyl compounds, such as crotonaldehyde and butenone (with the exception of butenols), as well as major secondary compounds, i.e., more than 90% of the output effluent associated with the process.
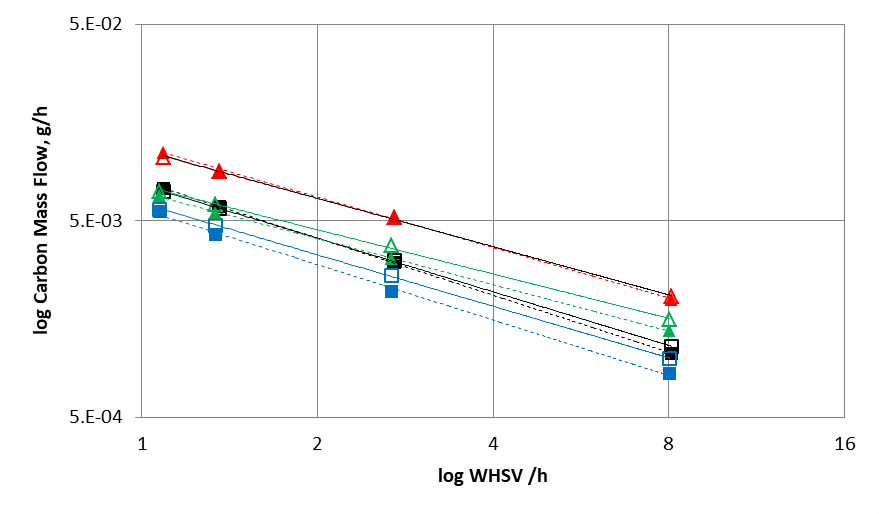
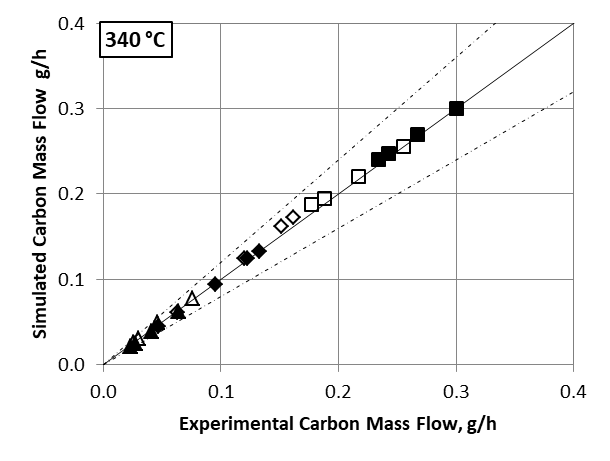
The development of this original kinetic model opens up new avenues. From a fundamental research point of view, ab initio calculations would lead to a greater understanding of reaction mechanisms on an atomic scale in order to test the hypothesis that the mono-site nature of adsorption does not make it possible to reproduce some inhibition effects observed experimentally.
From the point of view of applied research, the integration of this kinetic model within a complete reactor model, taking into account thermal and hydrodynamic effects, as well as the catalyst deactivation phenomenon, paves the way for an optimization of processes through numerical simulations.
The results of this research may now be used in applied projects targeting butadiene production with, in particular, the possibility, where a biobased feed is used, of sustainably producing elastomers for tires, sports items, or resins used in colored creative games pieces.
1 the first reactor is dedicated to the partial conversion of ethanol into acetaldehyde, via a dehydrogenation reaction, using a Cu/SiO2 catalyst, while the conversion of butadiene takes place in the second reactor, using a silica-supported tantalum oxide or similar as the catalyst, from a mixture {ethanol + acetaldehyde}, via a succession of chemical reactions: aldol condensation, Meerwein Ponndorff Verley reduction and dehydration.
2 WHSV (Weight Hourly Space Velocity): mass flow rate divided by the mass of the catalyst
This work has been realised within the phD work of Damien Dussol: New insights of butadiene production from ethanol: Elucidation of concurrent reaction pathways and kinetic study
References
[1] D. Dussol, N. Cadran, N. Laloue, L. Renaudot, J.-M. Schweitzer, New insights of butadiene production from ethanol: Elucidation of concurrent reaction pathways and kinetic study, Chem. Eng. J., 391 (2020) 123586
[2] Y.A. Gorin, Catalytic conversions of alcohols into bivinyl hydrocarbons. XV. General laws of formation of diethylenic hydrocarbons with a conjugate bond system, Russ. J. Gen. Chem. 19 (1949) 877–883.
[3] H. E. Jones, E. E. Stahly et B. B. Corson, Butadiene from alcohol. Reaction mechanism, Journal of American Chemical Society, vol. 71, pp. 1822-1828, 1949
[4] R. Inoue, A. Ichikawa, K. Furukawa, Synthesis of butadiene from 4-oxy-2-butanone and alcohol, J. Chem. Soc. Jpn. 61 (1958) 566–569.
Scientific contact : nicolas.laloue@ifpen.fr