To achieve the objectives of the energy transition, France has turned its attention to the production and use of low-carbon and renewable hydrogen. Mainly used until now in the chemicals and refining sectors, this energy vector may be useful to contribute to the decarbonization of some industrial sectors, for electricity storage or as a fuel in the transport sector. However, a number of challenges still need to be overcome before hydrogen technologies can be rolled out.
- Hydrogen: a market with significant potential
- The hydrogen value chain
- Hydrogen in the energy transition
- What future for hydrogen?
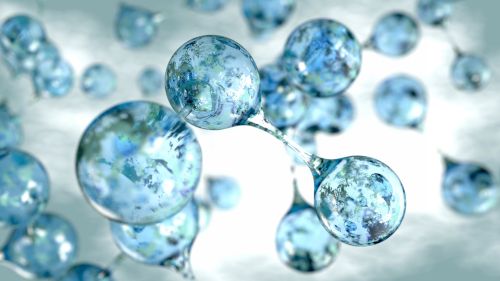
HYDROGEN A MARKET WITH SIGNIFICANT POTENTIAL
Hydrogen not only offers significant potential in terms of the reduction in greenhouse gases, but also major economic benefits. European countries are currently investing heavily in this rapidly-expanding market, starting with France where 100,000 new direct jobs are expected to be created (source: AFHYPAC). The country has already introduced a number of support initiatives (Investments for the Future Program, research support via the ANR, help for SMEs via the BPI, support for the roll-out of hydrogen mobility via Ademe, etc.) and set up a National Hydrogen Council. (Figure 1).
Figure 1 - National plans for hydrogen in France
THE HYDROGEN VAlUE CHAIN
What is hydrogen?
Like electricity, dihydrogen H2 (hydrogen) is primarily an energy vector and not an energy in itself, since it is produced as a result of a chemical reaction based on a primary resource.
Currently, for economic reasons, 95% of hydrogen is produced via the conversion of fossil energies, with almost half coming from natural gas.
Production involves extracting di-hydrogen H2 from the primary resource.
Where is hydrogen found?
The main resources exploited for di-hydrogen H2 production (referred to hereafter as hydrogen, despite this being technically incorrect) are water and hydrocarbons (coal, oil and gas).
• A single water molecule is made up of one atom of oxygen and two atoms of hydrogen, as reflected in the formula H2O.
• Hydrocarbons are produced by a combination of carbon and hydrogen atoms. Such is the case, for example, with methane, the principal component of natural gas, with a formula of CH4, one of the simplest combinations for hydrocarbons.
Hydrogen also exists in its natural state. The first natural sources of hydrogen were discovered in the depths of the seas in the 1970s and more recently on land. While knowledge about hydrogen has significantly progressed in recent years, there is still a need to assess the potential of this resource for the energy transition: What volumes currently exist? What are the potential reserves? What production methods are available? Similarly, understanding the mechanisms of formation, migration, and accumulation of hydrogen in the subsurface, which would enable more targeted exploration, still raises a number of questions. Scaling up industrial production by 2035/2040 requires heavier investment in research and development on this subject.
Read more about our expert advice in the field of hydrogen
How is hydrogen produced?
Different production methods exist:
- Steam natural gas reforming is the most widely employed technique. It consists in causing methane to react with water to obtain a mixture of hydrogen and CO2. The CO2 emitted by this process may potentially be captured and stored to produce low-carbon hydrogen. Instead of natural gas, the use of biomethane (methane produced via biomass fermentation) is another solution for producing low-carbon hydrogen.
- Hydrogen can also be produced from water and electricity via the electrolysis of water. The electrolyzer separates water into hydrogen and oxygen. At present, the method is not widely employed since it costs significantly more (2 to 3 times more expensive than natural gas reforming). It is reserved for specific uses that require a high degree of purity, such as electronics.
- Gasification involves using combustion to produce a mixture of CO and H2 from coal (a solution that emits high levels of CO2) or biomass.
«Today, 95% of hydrogen is produced from hydrocarbons (oil, natural gas and coal), the most profitable solution. However, this process emits CO2, a greenhouse gas. Industrial players are increasingly examining the possibility of producing hydrogen via electrolysis using low-carbon energies. But the obstacle remains the associated production costs, which are considerably higher than those of reforming.»
Guy Maisonnier, economic engineer, IFPEN
Green, gray, blue and yellow hydrogen: what are they?
- Green hydrogen is produced via the electrolysis of water using electricity generated uniquely from renewable energy;
- Gray hydrogen is produced via thermochemical processes using raw materials from fossil sources (coal or natural gas);
- Blue hydrogen is produced in the same way as gray hydrogen, the difference being that the CO2 emitted during production is captured for subsequent re-use or storage.
- Yellow hydrogen, more specific to France, is produced, like green hydrogen, via electrolysis but the electricity is primarily generated from nuclear energy.
Ademe recently suggested changing the terminology. Hydrogen previously referred to as “green” is now referred to as “renewable”, “gray” hydrogen becomes “fossil” and “blue” and “yellow” hydrogens are categorized as being “low-carbon”.
Once produced, the hydrogen must be stored and then transported to its place of distribution and use.
How is hydrogen stored?
Hydrogen has a very high specific energy (1 kg of hydrogen contains as much energy as around 3 kg of oil) but a very low volumetric density. It has to be converted to be able to store it in a volume that can be used:
- by compressing it to 700 bar: 7 liters of hydrogen can contain as much energy as 1 liter of gasoline;
- by liquefying it to compress it further by cooling it to a temperature of - 253°C: 4 liters of liquid hydrogen equate to 1 liter of gasoline.
Densifying hydrogen makes it possible to operate at lower pressures but demands more energy, making it most expensive.
Various storage options are available (batteries, mass storage in salt caverns) depending on the intended use.
How is hydrogen transported?
Hydrogen is generally transported in compressed form via a relatively extensive network of pipelines; a total of more than 4,500 km in the world, including 1,600 km in Europe and 2,500 km in the USA.
Countries like Japan are considering importing hydrogen, which would be shipped in from Australia, for example.
How is hydrogen currently used?
There are currently two principal uses of hydrogen: firstly, it is a base material for the production of ammonia (fertilizer) and methanol; secondly, it is used as a reagent in the refining processes that convert crudes into oil products, fuels and biofuels.
However, there are numerous other potential uses for the gas, and hydrogen is a promising solution for decarbonizing a number of industrial sectors and supporting the energy transition.
He had already thought of it
“Yes, my friends, I believe that water will one day be used as a fuel, that hydrogen and oxygen which constitute it, used singly or together, will furnish an inexhaustible source of heat and light, of an intensity of which coal is not capable”, wrote Jules Verne in his novel The Mysterious Island in 1874.
Figure 2 -The outlook for hydrogen
HYDROGEN IN THE ENERGY TRANSITION
Two methods are available for recovering the energy from previously stored renewable or low-carbon hydrogen:
- either in the form of heat via its direct combustion with oxygen;
- or in the form of electricity via a fuel cell.
In both cases the global reaction only produces water and the energy generated can be used for a variety of purposes. Hydrogen has been assigned three essential objectives for a successful energy transition.
Hydrogen to decarbonize the transport sector
In France, the transport sector is responsible for 27% of global greenhouse gas emissions, the highest figure of any sector. The stakes where mobility is concerned are therefore very high, since the hydrogen solution applied to clean mobility (power-to-mobility – Figure 3), using direct combustion or fuel cells, results in a significant reduction in emissions.
Hydrogen in the transport sector: a few figures
A diesel vehicle produces between 40 and 45 metric tons of CO2 over the course of its lifetime, a hydrogen vehicle produces just over 35 tons by reforming, and a hydrogen vehicle produces less than 15 tons by renewable electrolysis.
At best, hydrogen cars have a carbon impact that is 74% lower than conventional IC vehicles (Source: ARTS Carnot Institute).
The hydrogen vehicle
The hydrogen engine
The physicochemical characteristics of hydrogen make it a good candidate for use as a fuel in a “gasoline”-type spark ignition engine. The principal advantage lies in the environmental footprint: combined with oxygen, hydrogen combustion mainly produces water and heat, only emitting nitrogen oxides (NOx) in the process. However, this solution requires specific adaptations to obtain a high level of efficiency and ultra-low NOx emissions. In particular, there is a need to exploit the different properties of hydrogen, such as its fast-burning capacity in a lean mixture.
The use of hydrogen in an internal combustion engine may benefit from the latest advances in IC engine technology and combination with a hybrid powertrain. Hence using technologies that are more robust and mature than those currently employed for fuel cells, it would be possible to achieve an efficiency in excess of 50%. This could be a transition solution towards a fuel cell since it makes it possible to validate the entire hydrogen production and distribution chain using existing industrial production facilities.
The fuel cell in electric cars
For the long term, car manufacturers are also focusing on fuel cells as power generators for electric vehicles. In doing so, they are seeking to consolidate solutions for battery-powered electric vehicles, currently hampered by limited range and the time required to recharge the batteries. Hydrogen can be used as a fuel for a fuel cell — which produces electricity — to allow the electric motor to operate and propel the vehicle. Hydrogen is one of the best energy vectors for fuel cells today in terms of energy and emissions performances. Their overall efficiency is above 50% over a broad working range, representing a significant improvement compared to existing gasoline IC engines.
Powered by a combination of air and hydrogen, the battery converts the chemical energy from hydrogen into electric energy according to a reverse electrolysis principle. By causing hydrogen to react with oxygen on electrodes (thin membranes covered with a catalyst, platinum), fuel cells can be used to produce electricity, the only emission being steam. The principle dates back to 1839! It has long been used to generate electricity onboard rockets.
The hydrogen train and plane
One of the objectives of the French national hydrogen plan is the commissioning of the first hydrogen train in the country in 2022. Following Germany's lead, France is taking its first steps on the road to the use of hydrogen on its railways, with the SNCF and the Regions launching the TER H2 project aimed at introducing the first fleet of regional hydrogen trains in several pilot regions across the country.
The hydrogen plane or “clean plane”, while the focus of ambitious projects, still has a number of technological, environmental and safety questions to address.
Hydrogen for electricity storage and its injection into the networks
Energy storage in the form of hydrogen makes it possible to compensate for the intermittent nature of renewable energies (wind and solar) by optimizing electricity production capacity (power-to-power – Figure 3).
Within the framework of the development of a renewable energy mix, electrolysis makes it possible, when the network is in surplus (i.e., when electricity production exceeds consumption), to store hydrogen for a varying length of time depending on needs.
Conversely, when the network is in deficit, the hydrogen available can be reused in a fuel cell to produce electricity.
Hydrogen can also be injected directly into the gas networks (power-to-gas – Figure 3):
- via direct injection into gas networks for combustion;
- via synthetic methane production (using the methanation principle): conversion of carbon monoxide (CO) or carbon dioxide (CO2) in the presence of hydrogen, which can then be converted into heat, electricity or fuel.
Hydrogen can also be injected directly into the gas networks (power-to-gas – Figure 3):
- via direct injection into gas networks for combustion;
- via synthetic methane production (using the methanation principle): conversion of carbon monoxide (CO) or carbon dioxide (CO2) in the presence of hydrogen, which can then be converted into heat, electricity or fuel.
Hydrogen to decarbonize the transport sector
Hydrogen can used in the industrial sector (power-to-industry – Figure 3):
- firstly to provide low-carbon energy to the industrial units concerned;
- secondly to contribute to the decarbonization of the industrial processes concerned replacing the fossil energies currently used: one example is the manufacture of steel via iron ore reduction. Today this reduction process depends on coal but low-carbon hydrogen may be used in the future.
The roll-out of renewable hydrogen is on the horizon. Large-scale projects are being set up, such as NortH2, Europe’s biggest green hydrogen production project. Objective: to produce green hydrogen using renewable electricity from offshore wind turbines off the coast of the Netherlands.
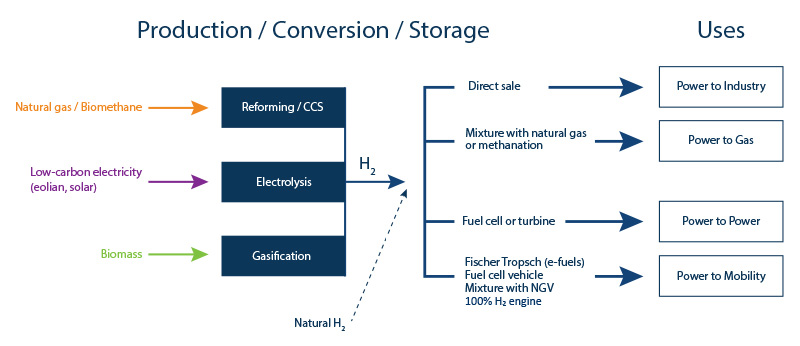
WHAT FUTURE FOR HYDROGEN?
The roll-out of low-carbon hydrogen can be envisaged by the end of this decade, with significant development likely in the next. To make this possible, a number of challenges need to be overcome.
Reduce costs
Green hydrogen is very expensive and can only be rolled out once costs across the entire value chain have been reduced, starting with the cost of producing renewable electricity (solar, wind) but also electrolyzer and fuel cell costs.
How much does low-carbon hydrogen cost?
The cost of producing hydrogen via electrolysis is currently two to three times higher than steam reforming and two times higher than reforming with CO2 capture. At present, this option is reserved for specific usages such as electronics, which demand a high degree of purity.
Nevertheless, these costs continue to fall and there is the genuine expectation that scale effects will lead to a situation whereby the costs of hydrogen produced via water electrolysis will be aligned with those of fossil hydrogen.
In addition, the complexity of the value chain and the different conversions imply efficiency cascades generating energy losses, thereby increasing production costs.
In parallel, a relatively high cost of CO2 would make it possible to reduce the cost difference with natural gas reforming. However, the increase in carbon tax should be progressive and go hand-in-hand with public policies to support the least well-off populations.
Build infrastructures
The roll-out of electrolytic hydrogen requires the introduction of a complex infrastructure incorporating, in addition to production capacities powered by renewable energies (wind and solar farms, connected or otherwise to the grid), a transport and distribution network connecting these production capacities to sites where it will be used, and a variety of networked storage facilities. The entire infrastructure will have to be managed by a smart system designed to optimize the matching of supply and demand over daily or temporary time periods.
It is also necessary to pool the construction of CO2 capture, transport and storage infrastructures. In Europe, the first such units will be operational by the middle of the decade.
The roll-out of transport and distribution infrastructures requires considerable investments and a relatively long implementation period.
POINTS TO REMEMBER
Low-carbon renewable hydrogen represents a market of significant potential. To exploit this potential, France is investing heavily in the sector within the framework of a structured national hydrogen policy.
Hydrogen is an energy vector that requires a complex value chain. Generally, it is produced from water or hydrocarbons, either via thermochemical processes with the capture of CO2 emitted during production, or via water electrolysis. Once stored and transported if necessary, it is used as energy with a fuel cell or via direct combustion.
As part of the energy transition, France aims to extend the use of low-carbon and renewable hydrogen to supply the transport sector, compensate for the intermittent nature of renewable energies via electricity storage, and decarbonize industry.
The roll-out of hydrogen technologies is still largely dependent on reducing their costs and infrastructure construction.